Method 2:
Single cell recording allows an investigator to record the activity of an individual neuron. Different techniques are available, but most commonly a rat (or cat, or other non-human animal) will undergo surgery under anesthesia that allows an anchoring device to be affixed to the skull. The device serves as a guide for a microelectrode to be placed in the brain region of interest. The microelectrode measures changes in electrical potential just outside a neuron--changes associated with an action potential. In other words, it measures each time the neuron "fires."
When the animal recovers from surgery, researchers can "eavesdrop" on the activity of individual neurons while the animal is awake and behaving.
The goal is to figure out what makes the neuron fire. The technique is to expose the animal to many different situations, and to note what makes the neuron you're recording from respond maximally.
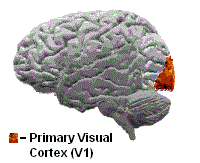
David Hubel and Torsten Wiesel performed exactly this experiment in the 1950s and reported that cells in primary visual cortex of cats responded maximally to simple lines of a particular orientations.
The result was a series of models in the early 1960s that used lines as the starting point for complex visual processing.
So another method of integrating neuroscientific data into behavioral theory is using data from single-cell recording studies to make an educated guess as to what the brain codes, and then using the guess as the foundational representation in a cognitive model.
How does this relate to education?
This technique is not often used, but one example might be John Stein's (2001) magnocelluar theory of dyslexia, which puts cells in the magnocellular layer of the lateral geniculate nucleus of the thalamus (click here for image) in a central role in dyslexia. These cells are crucial for timing of rapid events, including (Stein argues) for the stability of eye fixation when you move your eyes (including when you move your eyes as you read); hence, kids with dyslexia are more likely to have the image of letters slip out of the field of view, as well as other problems. In addition, they will have deficits in hearing that are also traceable to problems with precise timing, and these hearing problems also affect reading.
Stein's work has its roots in single cell recording work that first distinguished the role of cells in the magnocellular layer from those in the parvocellular layer (e.g., Derrington, 1984).
Yesterday we saw that neuroscientific data might provide a researcher with clues about the large-scale architecture supporting a cognitive process. Today we have moved to a much finer level of detail; instead of the overall plan, neuroscientific data provided hints about the nature of the building blocks.
Tomorrow, Method 3.
References:
Derrington , A. M . ( 1984 ). Spatial and temporal contrast sensitivities
of neurons in lateral geniculate nucleus of macaque . Journal of
Physiology, 357, 219 – 240
Selfridge, O. G. & Neisser, U. (1960), Pattern recognition by machine, Scientific American 203, 60-68
Stein, J. The magnocellular theory of developmental dyslexia. Dyslexia, 7, 12-36.